
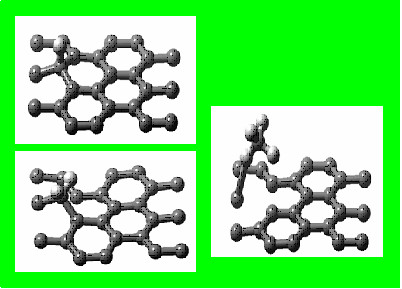
In addition, NMC-based cathodes can outperform lithium iron phosphate (LFP) cathodes in many areas, particularly in terms of operational voltage in which LFP-based LIBs can only output voltages below 3.4 V and suffer from high rates of self-discharge. As compared with LiMO 2 (M = Ni, Mn, or Co) cathodes, NMC-based cathodes also possess the combined merits of three transition metals in which nickel can offer high specific capacities, whereas Co and Mn can provide layered structures and enhanced structural integrity. Overall, the chemistries of LIBs are constantly evolving, and in terms of cathode materials, LiNi xMn 圜o 1− x− yO 2 (NMC) layered oxides have received increasing attention due to their enhanced specific capacity and thermal stability. Finally, this review will summarize current research focuses and propose future research directions.ĭue to significant advantages such as high energy densities, high galvanic potentials, wide temperature ranges, no memory effects and long service lifespans, lithium-ion batteries (LIBs) have been widely employed in various applications, including in the fields of communication, aviation and transportation. Furthermore, this review will present various physical and electrochemical diagnostic tools that are vital in the elucidation of degradation mechanisms during operation to supplement future degradation studies. Electrolyte components that can facilitate the stabilization of anodic solid-electrolyte interfaces (SEIs) are also reviewed and tradeoffs between modification techniques as well as controversies are discussed for a deeper understanding of the mitigation strategies of Ni-rich NMC/graphite LIBs. In addition, this review will categorize advanced mitigation strategies for both electrodes based on different modifications in which Ni-rich NMC cathode improvement strategies involve dopants, gradient layers, surface coatings, carbon matrixes and advanced synthesis methods, whereas graphite anode improvement strategies involve surface coatings, charge/discharge protocols and electrolyte volume estimations. And with a broad collection of proposed mechanisms on both atomic and micrometer scales, this review can supplement previous degradation studies of Ni-rich NMC batteries. Based on this, this review will summarize recently reported and widely recognized studies of the degradation mechanisms of Ni-rich NMC cathodes and graphite anodes. The demand for lithium-ion batteries (LIBs) with high mass specific capacities, high rate capabilities and longterm cyclabilities is driving the research and development of LIBs with nickel-rich NMC (LiNi xMn 圜o 1− x− yO 2, x ≥ 0.5) cathodes and graphite (Li xC 6) anodes. Finally, this review will summarize current research focuses and propose future research directions. Electrolyte components that can facilitate the stabilization of anodic solid electrolyte interfaces are also reviewed, and trade-offs between modification techniques as well as controversies are discussed for a deeper understanding of the mitigation strategies of Ni-rich NMC/graphite LIBs. The demand for lithium-ion batteries (LIBs) with high mass-specific capacities, high rate capabilities and long-term cyclabilities is driving the research and development of LIBs with nickel-rich NMC (LiNi xMn 圜o 1− x− yO 2, \(x \geqslant 0.5\)) cathodes and graphite (Li xC 6) anodes.
